Compared with traditional chiral crystalline materials formed with chiral space groups, chiral mesostructured inorganic materials can be formed with nanocrystals of achiral space groups via self-assembly, which exhibits unique physical and chemical chiral anisotropy properties due to crystal torsion and helical stacking. However, it is very difficult to analyze such structures. Spectroscopy and X-ray diffraction only display overall structural information, and scanning electron microscopy capable of revealing the chiral morphology of twist or helical stacking cannot determine the orientation of nanocrystals helically stacked at a small angle. Other techniques such as precession electron diffraction, electron backscatter diffraction and nano-beam diffraction are not suitable for the chiral mesostructured inorganic crystals formed with achiral space groups, although they can resolve the chiral crystals formed with chiral space groups. The current approach to determining the twisting chirality of chiral inorganic crystals is to manually tilt and rotate the goniometer stage to align the various crystal zone axes with the electron beam firstly and then calculate the corresponding angular deflection and pitch. However, it is still greatly challenging to synchronously determine the hierarchical chirality and to quantify and analyze the specific twist angle, rotation axis, stacking mode and other structural information in depth.
Professor Han Lu's team has recently proposed a general method for determining the hierarchical chiral mesostructures via 3D electron diffraction reconstruction. Under this approach, a complete set of 3D electron diffraction data is reconstructed from 2D electron diffraction patterns within the tilting and rotation range of the goniometer stage of a transmission electron microscope; the hierarchical chiral structure information of inorganic crystals is solved synchronously based on the corresponding relationship between the electronic diffraction patterns modulated in reciprocal space and the crystal structure of mesostructured materials in real space via the corresponding diffraction data processing and calculation methods. The relevant research outcomes were reported by publishing a paper titled Synchronous Quantitative Analysis of Chiral Mesostructured Inorganic Crystals by 3D Electron Diffraction Tomography inNature Communications(Nat. Commun., 2022, 13, 5718, DOI: 10.1038/s41467-022-33443-1), a renowned international journal.
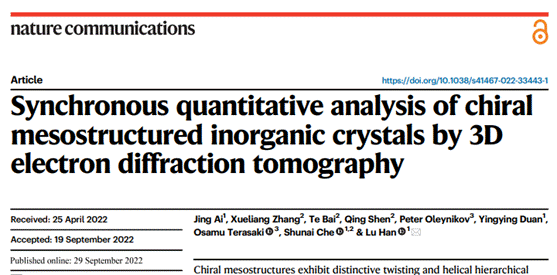
The researchers firstly built five typical crystal structural models and established the relationship model between the modulated structure in real space and the intensity distribution and relative position change of diffraction spots in reciprocal space by the Fourier transform. Single crystals are in independent diffractions arranged periodically in reciprocal space. Chiral mesostructures are in a diffraction plane corresponding to the crystal structure bending, with the diffraction spots in the intensity distribution of a "bending contour"; their diffraction is in a centrally symmetric arch-shaped intensity distribution consistent with the bending angle and direction of the crystals and shows helical characteristics. The helically stacked nanocrystals present a 3D electron diffraction dataset formed by helical stacking of multiple diffraction patterns. Therefore, the primary chiral torsion of mesoscopic crystal structures can be revealed by the intensity distribution shape of diffraction spots in the 3D reciprocal spatial lattice, and the relationship between the main diffraction spot and its surrounding satellite diffraction spots represents the second-level helical chirality of the nanocrystal stacking. According to the change of diffraction spots and the corresponding measured sample length, the torsion direction, pitch, hierarchical chirality and other information of chiral materials can be accurately calculated. Such information on chiral mesostructures hardly retrieved by the traditional electron microscopy techniques can be observed in the reconstructed 3D reciprocal space.
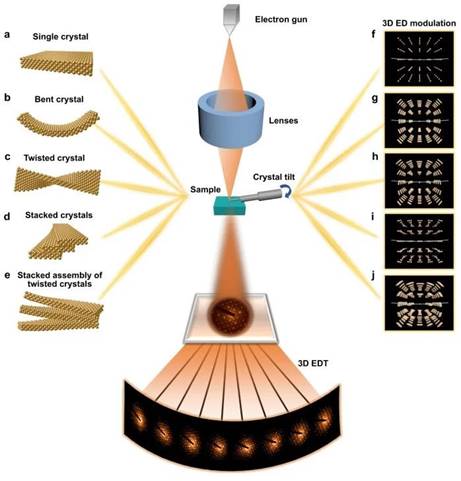
The researchers applied this method to chiral mesostructured nickel molybdate (L/D-CNM) hydrothermally synthesized from chiral molecule L/D-threonine as the symmetry-breaking agent and structure-directing agent, Fe2SO4•7H2O as the inorganic precursor, and successfully analyzed the first-level chirality of D-CNM lattice torsion through periodic arc-shaped diffraction by 3D electron diffraction and helical arrangement by vertical diffraction, and determined the central rotational axis of D-CNM as the normal direction of the (20-4) lattice plane. The D-CNM torsion angle was determined as 25.1° and the pitch length as about 33.0 μm, by the average central angle of the arc diffraction of the 3D electron diffraction patterns at both ends of the D-CNM rod-like particles perpendicular to the central axis of rotation.
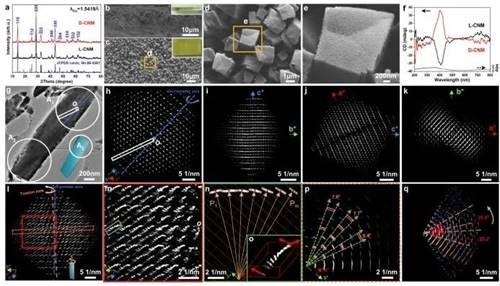
The primary chirality of lattice twisting and secondary chirality of helical stacking of chiral mesostructured tin dioxide crystals (L-CTD) synthesized with L-ammonium tartrate were also revealed by this method. The central axis of rotation of L-CTD was determined as the normal direction of the (1-10) lattice plane, the torsion angle as 2.2° and thepitch length[71]as about 26.2 μm. The second-level chirality of the helically stacked nanosheets in the L-CTD nanoplates was found by a series of diffraction spots stacked around the origin in slices cut from the 3D electron diffraction dataset. The dihedral angle of rotation of the nanosheets was determined as 1.3° by the average rotational angle of the diffraction.
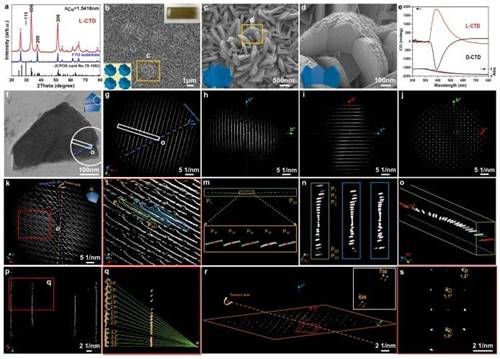
Finally, the correctness and validity of the 3D electron diffraction reconstruction approach in judging the hierarchical chiral self-assembles were verified by calculation and simulation. This research explored the application of quantitative analysis via 3D electron diffraction in the structural analysis of different types of chiral mesostructured crystals, providing a new idea for the fundamental understanding of the structure-activity relationship of materials and the construction and structural research of chiral inorganic materials in the future. In addition, this structural relationship and analytical method can be extended to self-assembled structures of various flexure crystals, defective crystals and nanocrystal stacking, which is expected to drive the further development in characterization techniques of materials and has important research value for the science of chirality, the chemistry of nanomaterials and crystallography.
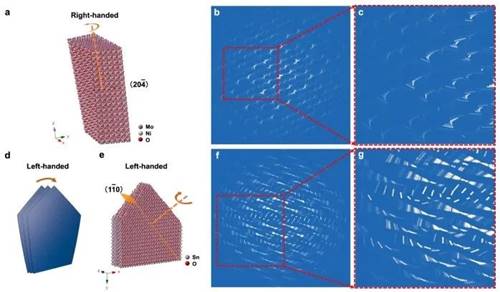
Professor Han Lu and Ai Jing, a doctoral candidate of our school contributed as the corresponding author and the first author of this paper respectively. Their research work was backed by the team of Professor Che Shunai and the teams of Professor Osamu Terasaki and Professor Peter Oleynikov from ShanghaiTech University. The research work was financially supported by the National Natural Science Foundation of China, the Special Fund for Basic Scientific Research Business Expenses of Central Colleges and Universities, the National Key R&D Program of China, the Science Fund of Science and Technology Commission of Shanghai Municipality and a funding project of the Research Center for High Resolution Electron Microscope of ShanghaiTech University.
Paper link:https://www.nature.com/articles/s41467-022-33443-1
Latest News: Breakthrough Made by Han Lu's Research Team in Mechanical Behaviors Regulation of Triply Periodic Minimal Surface Structures with Crystal Twinning, with Relevant Outcomes Published inAdditive Manufacturing.
Triply periodic minimal surface (TPMS) structures are excellent mechanical materials with high specific strength, high energy absorption and unique layered deformation mechanism, and have been applied in some important fields, including aviation, automobile, medical equipment and infrastructure. Due to their saddle shape-surface with non-positive Gaussian curvature, stress on the structures is divided into multiple components in different directions, and the structure is morerigidattributing to the stress deconcentration. However, the performance of TPMS structures is limited by anisotropic mechanical behavior owing to the oblique shear band with stress concentration when the load is beyond the yield stress, resulting in structural catastrophic failure.
Professor Han Lu's research team has recently proposed a strategy to manipulate the stress transfer path by introducing crystal twinning, which applies microscopic crystal structures in the design of macroscopic mechanical materials, realizing the deformation programmable TPMS structures, providing a new idea for structure design and protection. Their research outcomes were published in a paper titled Mechanical behaviors regulation of triply periodic minimal surface structures with crystal twinning online inAdditive Manufacturing(Additive Manufacturing 58 (2022) 103036).
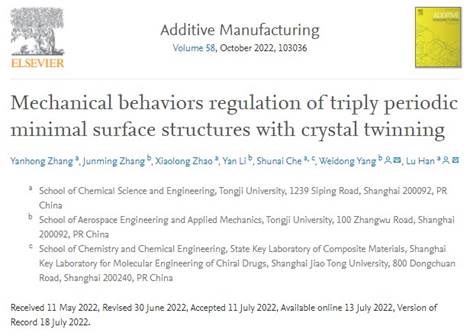
Crystal twinning is a common form of crystal defect consisting of two or more adjacent fragments of the same structure with an epitaxial orientation to each other, found in various biological and synthetic systems. Inspired by microscopic crystal defects, the team constructed crystal twin structures of gyroid and diamond, including single and multiple twin-related structures at different locations, and performed compression tests from various directions. The regulation and improvement of the bearing capacity and compressive deformation mechanism of the structures depend on the load transfer between the structural geometries. The original stress transfer path is blocked near the twin boundary due to the change of curvature, and the stress on the new structure is divided into equal components symmetrically due to the symmetry effect, so that the structure exhibits deformation shear bands symmetrical along the twin boundary, to effectively avoid the oblique shear band with stress concentration, thus protecting the structure from destructive collapse. Moreover, there is only a small fluctuation of Gaussian curvature at the twin boundary due to the smooth connection, which well retains the structural characteristics of the negative Gaussian curvature surface. Therefore, twinning has no great influence on the stress-strain behavior of structures. In addition, the introduction of a different number of twin boundaries into structures and the application of different loading directions can result in more varied deformation behaviors. The results from finite element simulation were consistent with the experimental results. Leveraging the advantages of the improved deformation mechanism, eliminated stress concentration and effectively enhanced structural integrity under a compression load in addition to retaining the mechanical properties of structures, this approach is expected to have a broad application prospect in directional blasting of buildings, human body protection devices and flexible electronics.
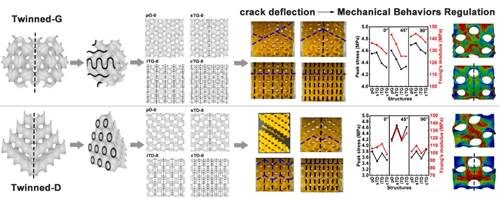
Professor Han Lu of our school and Professor Yang Weidong from the School of Aerospace Engineering and Applied Mechanics contributed as the corresponding authors of the paper, and Zhang Yanhong, a doctoral candidate of our school, and Zhang Junming, a doctoral candidate from the School of Aerospace Engineering and Applied Mechanics, as the co-first authors of the paper. The research work has been financially supported by the National Natural Science Foundation of China, the Fundamental Research Funds for the Central Universities, the Specially-Appointe Professor Program for Universities and Colleges of Shanghai Municipality, and the Shanghai Pujiang Program.